Nestled between four of the five Great Lakes, Michigan is a magnet for water recreation and manufacturing. With its diverse industries, the state has been also been a front-runner in response to PFAS contamination, a class of toxic, pervasive compounds known as “forever chemicals”. Comprehensive surveys have identified nearly 300 sites with detectable PFAS in various water sources throughout the state. Scientists at Michigan State University (MSU) are seeking solutions.
PFAS are as indestructible as diamonds are forever. They are built upon molecules that make them as valuable as glittering jewels, but far more devastating. Known scientifically as per- and polyfluoroalkyl compounds, PFAS have such strong carbon-fluorine bonds and amazing qualities that industry has developed over 12,000 varieties, used in everything from waterproofing to fire retardants to pharmaceuticals.
The same qualities that make these chemicals useful also make them harmful. Because they do not degrade naturally, every molecule ever produced is still with us, causing damage when they penetrate and inhabit our cells. Since first being synthesized in the 1930s for non-stick coatings like Teflon, PFAS chemicals have spread everywhere, accumulating in our water, air, soil, and bodies
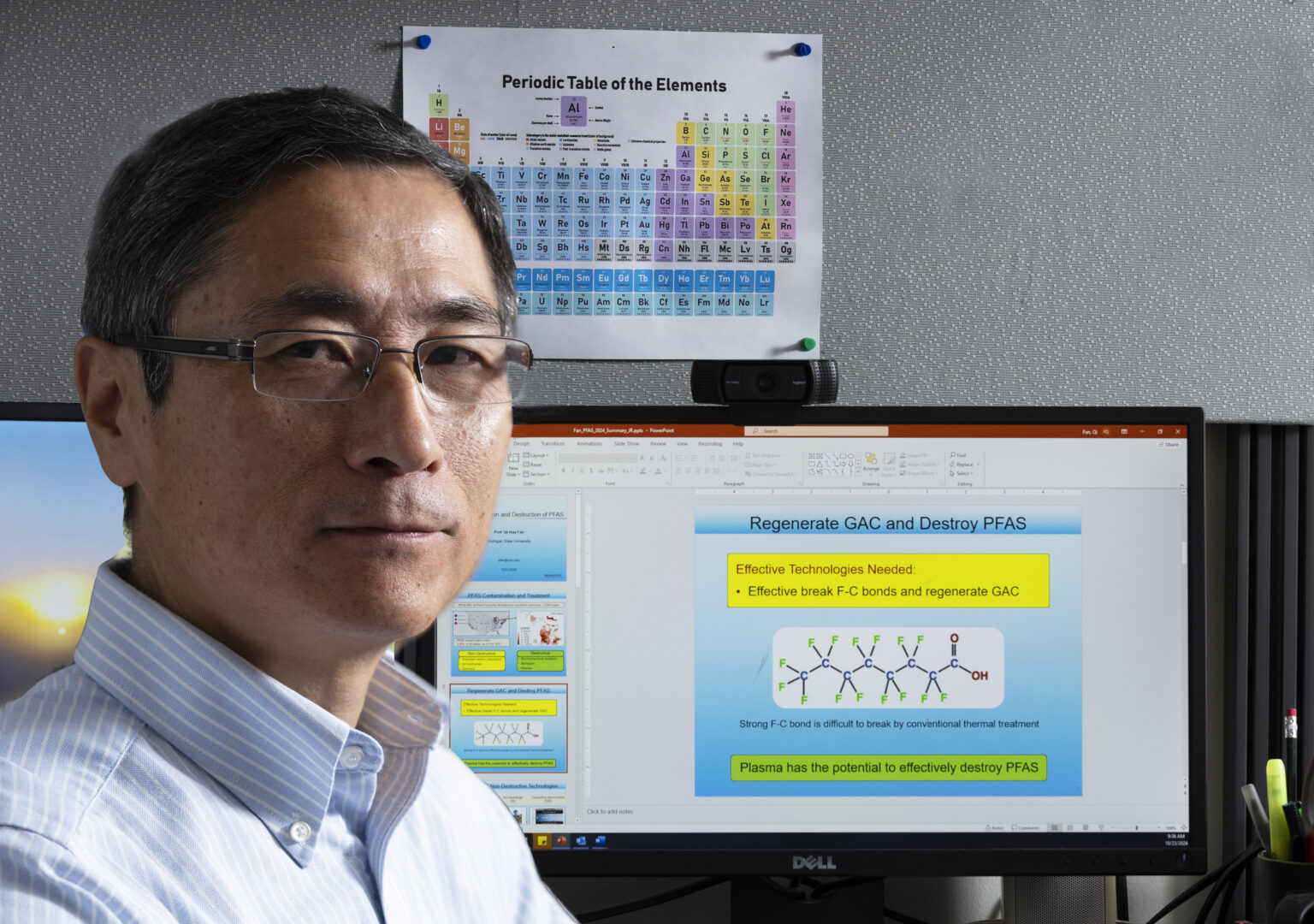
As early as the 1970s, manufacturers began linking PFAS to birth defects in children born of employees handling the chemicals. Epidemiologists have since traced PFAS to thyroid disease, liver damage, decreased fertility, and kidney and testicular cancer. Human cell membranes have both hydrophobic and hydrophilic components (water repulsive and water attractive), making them attractive homes for PFAS compounds that readily bind to proteins, disrupting the regulation of genes.
Last April, the EPA began requiring that water treatment facilities monitor six particularly harmful PFAS varieties and start removing them by 2029. Racing against the EPA clock, MSU and Fraunhofer USA – a subsidiary of Europe’s largest applied research organization with an institute on the MSU campus – are scoring impressive gains against PFAS.
“The PFAS problem is so big and it requires effort from many different directions,” says Qi Hua Fan, an MSU plasma physicist whose research is supported by the Great Lakes Protection Fund.
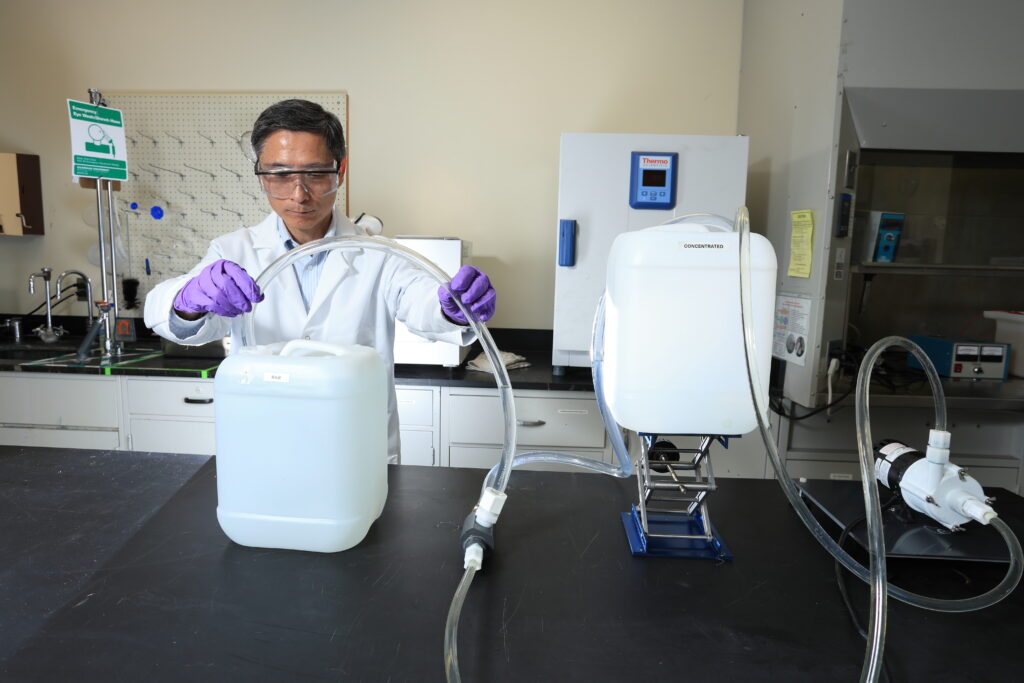
Current PFAS treatments remove contaminants from water by filtration, adsorption (collecting molecules on adsorbent surfaces), and reverse osmosis, but do not destroy them. This creates solid waste and wastewater with highly-concentrated PFAS that are often stored on industrial sites or in landfills where they remain a threat. Fan and his colleagues are tackling this threat with innovative combinations of plasma, granular activated carbon derived from biochar, micro- and nano-bubbles, and diamonds.
Plasma Magic
Plasma is everywhere – the fourth state of matter after solids, liquids, and gases – from lightning to northern light to fluorescent tubes. Plasma is in fact the most abundant form of ordinary matter in the universe, existing as clouds consisting of positively charged ions and negatively charged electrons, found mostly in stars (including the Sun).
The plasma encountered in routine life is called “cold plasma” or “low-temperature plasma”, and it can be generated by everyday electricity. A unique property of low-temperature plasma is many gaseous species co-exist and they have very different energies – the electrons are highly energetic with an average equivalent temperature in the order of 60,000 Fahrenheit degrees, and the ions and other gas species are much less energetic with an equivalent temperature ranging from tens to a few hundred degrees. Fan compares it to aluminum foil in a hot oven.
“You can touch the foil without getting burnt,” says Fan, “but not the pan. Why? Because it has very little mass. The foil’s heat capacity is small, but the temperature is very high. That’s how cold plasma acts – electrons are very energetic, but the gas is not.”
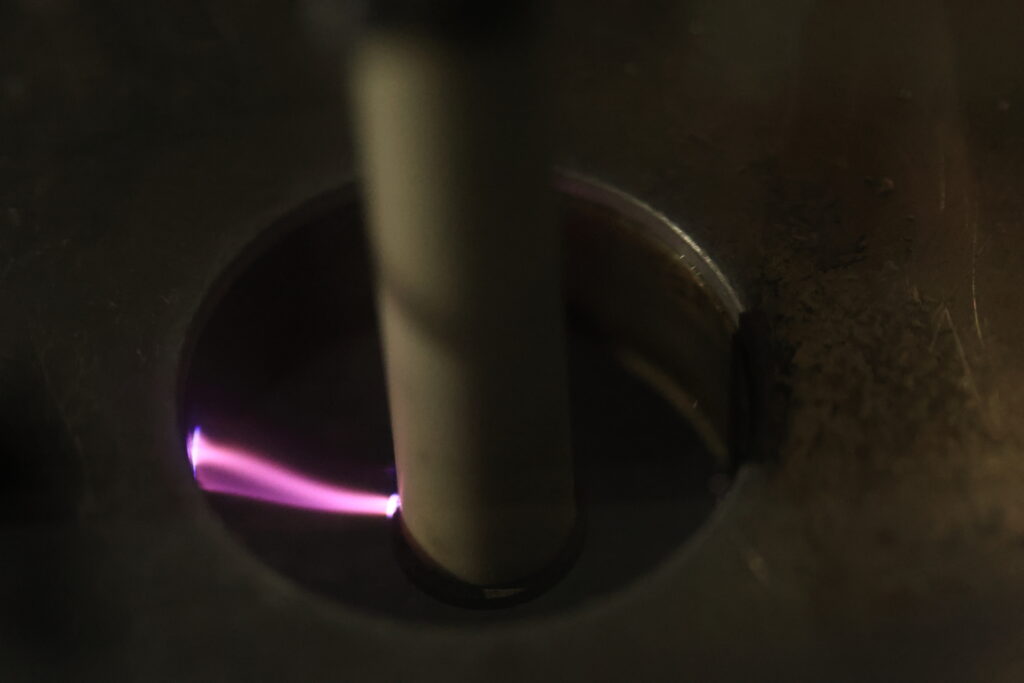
Low temperatures translate to low energy demands. That is the magic of cold plasma. Energy can be measured as electron volts or temperature. The carbon-fluorine bond in PFAS amounts to a few electron volts, a single electron volt equals over 20,000 degrees Fahrenheit.
“That’s a very strong bond,” he says, “and that’s why simply burning PFAS is hard to destroy them and would take so much energy. In an electric field, however, electrons can easily be accelerated to that same energy with just 100 volts, like a 50-watt light bulb, and can break apart PFAS bonds.”
When Fan launched his research seven years ago, he started with granular activated carbon (GAC) to remove PFAS found in low concentrations such as groundwater. GAC is a low-cost material made from carbon-rich biomass like wood and corn stalks. These biomass sources are sustainable and environmentally friendly. GAC can have a large surface area with a microporous structure. Just ten grams (about a third of an ounce) has the surface area of an entire football field. When water passes through it, contaminants stick to its ample surfaces.
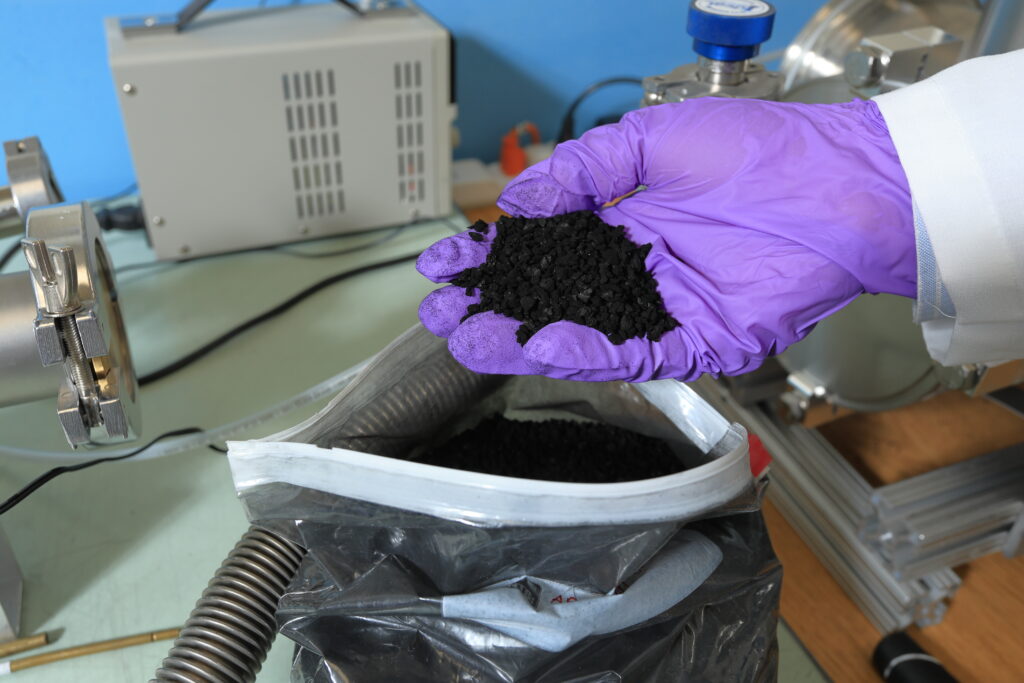
Using GAC to capture PFAS is particularly suitable for treating large volumes of water with low contaminant concentrations, such as drinking water and groundwater. The used GAC must be regenerated by destroying the adsorbed PFAS. Otherwise, it will cause recontamination if disposed of in landfills. Fan estimates the annual need for GAC to treat drinking water alone would ramp up to over 40 million lbs as the EPA regulation becomes effective. Hence, effectively regenerating GAC becomes a subsequent challenge. Fan and his team have devised a solution.
He developed a plasma system for regenerating GAC in what he calls a rotary reactor with fins (tumblers) that transport granules upward and simultaneously transverse, bathing them in plasma as they fall from top to bottom. By contrast, says Fan, burning GAC could worsen the problem, scattering intact PFAS into the air as a vapor and eventually getting into water and soil.
Glittering Successes
Fan’s patented rotary plasma reactor technology was a multi-faceted triumph. It captured, concentrated, and destroyed PFAS while reclaiming the valuable carbon – all with a minimum of energy and expense. But Fan had another target on his radar: industrial wastewater and landfill leachates with high PFAS concentrations; these water sources are usually too dirty for GAC adsorption due to clogging.
For this, he turned to direct plasma treatment of water in combination with micro- and nano-bubble generation and collection.
“For the Great Lakes Protection Fund project, we developed direct plasma destruction of concentrated PFAS in water,” says Fan, explaining that industrial wastewater and leachates would be the target applications.
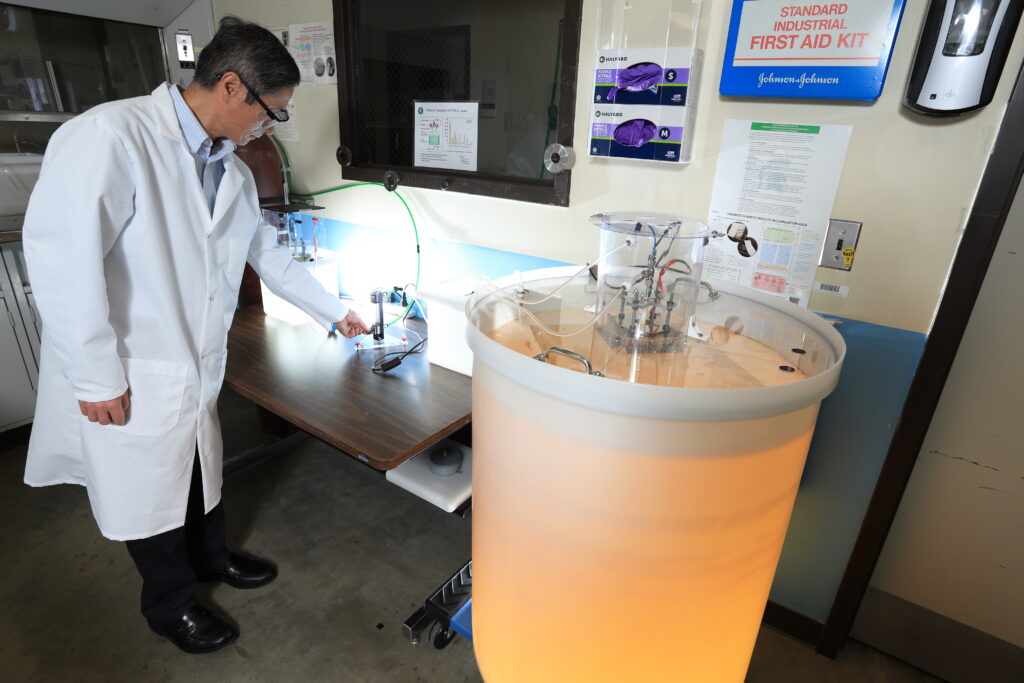
Donning a white coat and goggles, Fan proudly demonstrates his direct plasma prototype: a 100-gallon drum filled with amber liquid replicating industrial wastewater laden with salts, particulates, and PFAS. When he turns the system on, compressed air blown at high velocity enters a device at the bottom of the drum generating a myriad of bubbles that percolate upward in a thick foam loaded with pollutants. At the surface, an electrode surrounded by magnets creates a purple beam like a lighthouse gone amok.
“PFAS is carried by the foam into the plasma region where the plasma breaks the carbon-fluoride bonds,” says Fan.
The magnets enhance and strengthen the plasma with relatively low energy input while different surfactants added to the mix help snag diverse varieties of PFAS. Fan’s direct plasma system captures and destroys a variety of PFAS regulated by the EPA, but he cautions that his two technologies are suitable for specific applications – they are not silver bullets and no single approach will solve all situations.
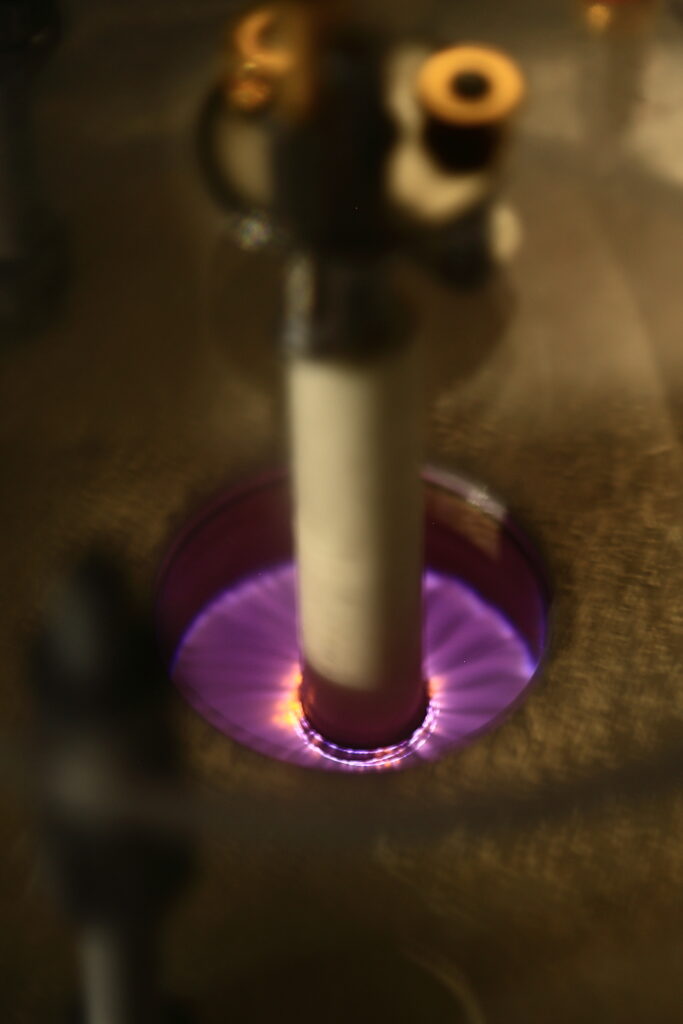
Down the hall in another lab, chemist Michael Becker of Fraunhofer USA has been working with Fan for years. With 30,000 employees worldwide, Fraunhofer partners with institutions of higher learning translating promising technologies by bridging the gap between academic discovery and industrial innovation.
“Part of our work here at MSU involves creating carbon materials with chemical vapor deposition,” says Becker, “and that includes boron-doped diamond electrodes. That is where the link with PFAS comes in.”
The boron-doped diamond electrodes engineered by Fraunhofer are particularly effective against PFAS through an electrochemical process. They create an abundance of hydroxyl radicals – highly reactive to attack the carbon-fluorine bonds in PFAS.
There are other electrode materials out there, Becker says, but they are less effective and stable than boron-doped diamonds. His partnership with Fan lends a certain irony to the PFAS saga. Using diamond electrodes to quash forever chemicals, he has shown that diamonds are not a forever chemical’s best friend.
Looking ahead, Fan says he has two goals. One is to cut PFAS contamination at its source by working with industry to reduce wastewater. And the second, where groundwater is already contaminated, is to apply his two technologies to remove and destroy PFAS.
“The Great Lakes Protection Fund project allowed us to develop scalable technologies to treat PFAS. Now we are at a stage to work with industry partners to use these technologies to solve real-world problems.”
Story and photos by Randall Hyman